The Slow Dynamics of Intracellular Sodium Concentration Increase the Time Window of Neuronal Integration: A Simulation Study
- PMID: 28970791
- PMCID: PMC5609115
- DOI: 10.3389/fncom.2017.00085
The Slow Dynamics of Intracellular Sodium Concentration Increase the Time Window of Neuronal Integration: A Simulation Study
Abstract
Changes in intracellular Na+ concentration ([Na+]i) are rarely taken into account when neuronal activity is examined. As opposed to Ca2+, [Na+]i dynamics are strongly affected by longitudinal diffusion, and therefore they are governed by the morphological structure of the neurons, in addition to the localization of influx and efflux mechanisms. Here, we examined [Na+]i dynamics and their effects on neuronal computation in three multi-compartmental neuronal models, representing three distinct cell types: accessory olfactory bulb (AOB) mitral cells, cortical layer V pyramidal cells, and cerebellar Purkinje cells. We added [Na+]i as a state variable to these models, and allowed it to modulate the Na+ Nernst potential, the Na+-K+ pump current, and the Na+-Ca2+ exchanger rate. Our results indicate that in most cases [Na+]i dynamics are significantly slower than [Ca2+]i dynamics, and thus may exert a prolonged influence on neuronal computation in a neuronal type specific manner. We show that [Na+]i dynamics affect neuronal activity via three main processes: reduction of EPSP amplitude in repeatedly active synapses due to reduction of the Na+ Nernst potential; activity-dependent hyperpolarization due to increased activity of the Na+-K+ pump; specific tagging of active synapses by extended Ca2+ elevation, intensified by concurrent back-propagating action potentials or complex spikes. Thus, we conclude that [Na+]i dynamics should be considered whenever synaptic plasticity, extensive synaptic input, or bursting activity are examined.
Keywords: mitral cells; neuronal modeling; purkinje cells; pyramidal cells; sodium dynamics; sodium-calcium exchanger; sodium-potassium-exchanging ATPase.
Figures
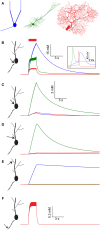
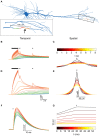

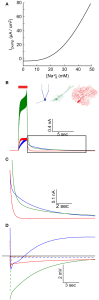
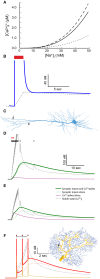
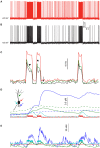
Similar articles
-
Synchronous Infra-Slow Bursting in the Mouse Accessory Olfactory Bulb Emerge from Interplay between Intrinsic Neuronal Dynamics and Network Connectivity.J Neurosci. 2017 Mar 8;37(10):2656-2672. doi: 10.1523/JNEUROSCI.3107-16.2017. Epub 2017 Feb 1. J Neurosci. 2017. PMID: 28148726 Free PMC article.
-
Na+ microdomains and sparks: Role in cardiac excitation-contraction coupling and arrhythmias in ankyrin-B deficiency.J Mol Cell Cardiol. 2019 Mar;128:145-157. doi: 10.1016/j.yjmcc.2019.02.001. Epub 2019 Feb 5. J Mol Cell Cardiol. 2019. PMID: 30731085
-
Mechanisms of neuronal hyperexcitability caused by partial inhibition of Na+-K+-ATPases in the rat CA1 hippocampal region.J Neurophysiol. 2002 Dec;88(6):2963-78. doi: 10.1152/jn.00244.2002. J Neurophysiol. 2002. PMID: 12466422
-
Role of intracellular sodium overload in the genesis of cardiac arrhythmias.J Cardiovasc Electrophysiol. 1997 Jun;8(6):700-21. doi: 10.1111/j.1540-8167.1997.tb01834.x. J Cardiovasc Electrophysiol. 1997. PMID: 9209972 Review.
-
The Na+/K(+)-pump in rat peritoneal mast cells: some aspects of regulation of activity and cellular function.Dan Med Bull. 1995 Nov;42(5):441-54. Dan Med Bull. 1995. PMID: 8747801 Review.
Cited by
-
An electrodiffusive network model with multicompartmental neurons and synaptic connections.PLoS Comput Biol. 2024 Nov 12;20(11):e1012114. doi: 10.1371/journal.pcbi.1012114. eCollection 2024 Nov. PLoS Comput Biol. 2024. PMID: 39531480 Free PMC article.
-
Slow G-Quadruplex Conformation Rearrangement and Accessibility Change Induced by Potassium in Human Telomeric Single-Stranded DNA.J Phys Chem B. 2024 Jun 27;128(25):5950-5965. doi: 10.1021/acs.jpcb.4c00719. Epub 2024 Jun 14. J Phys Chem B. 2024. PMID: 38875355
-
Uncertainty quantification and sensitivity analysis of neuron models with ion concentration dynamics.PLoS One. 2024 May 21;19(5):e0303822. doi: 10.1371/journal.pone.0303822. eCollection 2024. PLoS One. 2024. PMID: 38771746 Free PMC article.
-
Stimulus-Induced Theta-Band LFP Oscillations Format Neuronal Representations of Social Chemosignals in the Mouse Accessory Olfactory Bulb.J Neurosci. 2023 Dec 13;43(50):8700-8722. doi: 10.1523/JNEUROSCI.1055-23.2023. J Neurosci. 2023. PMID: 37903594 Free PMC article.
-
Temporal dynamics of Na/K pump mediated memory traces: insights from conductance-based models of Drosophila neurons.Front Neurosci. 2023 May 22;17:1154549. doi: 10.3389/fnins.2023.1154549. eCollection 2023. Front Neurosci. 2023. PMID: 37284663 Free PMC article.
References
LinkOut - more resources
Full Text Sources
Other Literature Sources
Molecular Biology Databases
Research Materials
Miscellaneous