Allosteric regulation of catalytic activity: Escherichia coli aspartate transcarbamoylase versus yeast chorismate mutase
- PMID: 11528003
- PMCID: PMC99034
- DOI: 10.1128/MMBR.65.3.404-421.2001
Allosteric regulation of catalytic activity: Escherichia coli aspartate transcarbamoylase versus yeast chorismate mutase
Abstract
Allosteric regulation of key metabolic enzymes is a fascinating field to study the structure-function relationship of induced conformational changes of proteins. In this review we compare the principles of allosteric transitions of the complex classical model aspartate transcarbamoylase (ATCase) from Escherichia coli, consisting of 12 polypeptides, and the less complicated chorismate mutase derived from baker's yeast, which functions as a homodimer. Chorismate mutase presumably represents the minimal oligomerization state of a cooperative enzyme which still can be either activated or inhibited by different heterotropic effectors. Detailed knowledge of the number of possible quaternary states and a description of molecular triggers for conformational changes of model enzymes such as ATCase and chorismate mutase shed more and more light on allostery as an important regulatory mechanism of any living cell. The comparison of wild-type and engineered mutant enzymes reveals that current textbook models for regulation do not cover the entire picture needed to describe the function of these enzymes in detail.
Figures

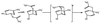
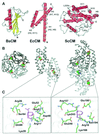


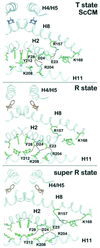
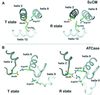
Similar articles
-
Allostery and cooperativity in Escherichia coli aspartate transcarbamoylase.Arch Biochem Biophys. 2012 Mar 15;519(2):81-90. doi: 10.1016/j.abb.2011.10.024. Epub 2011 Dec 16. Arch Biochem Biophys. 2012. PMID: 22198283 Free PMC article. Review.
-
Mechanisms of catalysis and allosteric regulation of yeast chorismate mutase from crystal structures.Structure. 1997 Nov 15;5(11):1437-52. doi: 10.1016/s0969-2126(97)00294-3. Structure. 1997. PMID: 9384560
-
Structure and mechanisms of Escherichia coli aspartate transcarbamoylase.Acc Chem Res. 2012 Mar 20;45(3):444-53. doi: 10.1021/ar200166p. Epub 2011 Oct 19. Acc Chem Res. 2012. PMID: 22011033 Free PMC article. Review.
-
Direct structural evidence for a concerted allosteric transition in Escherichia coli aspartate transcarbamoylase.Nat Struct Biol. 2001 May;8(5):423-6. doi: 10.1038/87582. Nat Struct Biol. 2001. PMID: 11323717
-
Divergent allosteric patterns verify the regulatory paradigm for aspartate transcarbamylase.J Mol Biol. 1999 Dec 17;294(5):1387-400. doi: 10.1006/jmbi.1999.3315. J Mol Biol. 1999. PMID: 10600393
Cited by
-
Adaptive evolution in the Saccharomyces kudriavzevii Aro4p promoted a reduced production of higher alcohols.Microb Biotechnol. 2022 Dec;15(12):2958-2969. doi: 10.1111/1751-7915.14154. Epub 2022 Oct 28. Microb Biotechnol. 2022. PMID: 36307988 Free PMC article.
-
Genomic Insights Into Plant-Growth-Promoting Potentialities of the Genus Frankia.Front Microbiol. 2019 Jul 4;10:1457. doi: 10.3389/fmicb.2019.01457. eCollection 2019. Front Microbiol. 2019. PMID: 31333602 Free PMC article.
-
Expression, purification, crystallization and preliminary X-ray crystallographic studies of a cold-adapted aspartate carbamoyltransferase from Moritella profunda.Acta Crystallogr Sect F Struct Biol Cryst Commun. 2005 Mar 1;61(Pt 3):279-81. doi: 10.1107/S174430910500285X. Epub 2005 Feb 12. Acta Crystallogr Sect F Struct Biol Cryst Commun. 2005. PMID: 16511017 Free PMC article.
-
Evolution of 3-deoxy-D-arabino-heptulosonate-7-phosphate synthase-encoding genes in the yeast Saccharomyces cerevisiae.Proc Natl Acad Sci U S A. 2005 Jul 12;102(28):9784-9. doi: 10.1073/pnas.0504238102. Epub 2005 Jun 29. Proc Natl Acad Sci U S A. 2005. PMID: 15987779 Free PMC article.
-
Engineering Allostery into Proteins.Adv Exp Med Biol. 2019;1163:359-384. doi: 10.1007/978-981-13-8719-7_15. Adv Exp Med Biol. 2019. PMID: 31707711 Free PMC article. Review.
References
-
- Allewell N M. Escherichia coli aspartate transcarbamoylase: structure, energetics, and catalytic and regulatory mechanisms. Annu Rev Biophys Biophys Chem. 1989;18:71–92. - PubMed
-
- Andrews P R, Smith G D, Young I G. Transition-state stabilization and enzymic catalysis. Kinetic and molecular orbital studies of the rearrangement of chorismate to prephenate. Biochemistry. 1973;12:3492–3498. - PubMed
-
- Aucoin J M, Pishko E J, Baker D P, Kantrowitz E R. Engineered complementation in Escherichia coli aspartate transcarbamoylase. Heterotropic regulation by quaternary structure stabilization. J Biol Chem. 1996;271:29865–29869. - PubMed
-
- Baker D P, Stebbins J W, DeSena E, Kantrowitz E R. Glutamic acid 86 is important for positioning the 80's loop and arginine 54 at the active site of Escherichia coli aspartate transcarbamoylase and for the structural stabilization of the C1–C2 interface. J Biol Chem. 1994;269:24608–24614. - PubMed
-
- Bartlett P A, Johnson C R. An inhibitor of chorismate mutase resembling the transition-state conformation. J Am Chem Soc. 1985;107:7792–7793.
Publication types
MeSH terms
Substances
LinkOut - more resources
Full Text Sources
Molecular Biology Databases