Single-cell transcriptomics of human-skin-equivalent organoids
- PMID: 37195865
- PMCID: PMC10348600
- DOI: 10.1016/j.celrep.2023.112511
Single-cell transcriptomics of human-skin-equivalent organoids
Abstract
Several methods for generating human-skin-equivalent (HSE) organoid cultures are in use to study skin biology; however, few studies thoroughly characterize these systems. To fill this gap, we use single-cell transcriptomics to compare in vitro HSEs, xenograft HSEs, and in vivo epidermis. By combining differential gene expression, pseudotime analyses, and spatial localization, we reconstruct HSE keratinocyte differentiation trajectories that recapitulate known in vivo epidermal differentiation pathways and show that HSEs contain major in vivo cellular states. However, HSEs also develop unique keratinocyte states, an expanded basal stem cell program, and disrupted terminal differentiation. Cell-cell communication modeling shows aberrant epithelial-to-mesenchymal transition (EMT)-associated signaling pathways that alter upon epidermal growth factor (EGF) supplementation. Last, xenograft HSEs at early time points post transplantation significantly rescue many in vitro deficits while undergoing a hypoxic response that drives an alternative differentiation lineage. This study highlights the strengths and limitations of organoid cultures and identifies areas for potential innovation.
Keywords: CP: Stem cell research; differentiation; human epidermis; hypoxia; organoid; single-cell RNA sequencing.
Copyright © 2023 The Author(s). Published by Elsevier Inc. All rights reserved.
Conflict of interest statement
Declaration of interests The authors declare no competing interests.
Figures
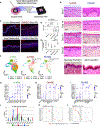




Similar articles
-
Human skin equivalents cultured under hypoxia display enhanced epidermal morphogenesis and lipid barrier formation.Sci Rep. 2019 May 24;9(1):7811. doi: 10.1038/s41598-019-44204-4. Sci Rep. 2019. PMID: 31127151 Free PMC article.
-
Integration of transcriptomics and spatial biology analyses reveals Galactomyces ferment filtrate promotes epidermal interconnectivity via induction of keratinocyte differentiation, proliferation and cellular bioenergetics.Int J Cosmet Sci. 2024 Dec;46(6):927-940. doi: 10.1111/ics.12991. Epub 2024 Jun 24. Int J Cosmet Sci. 2024. PMID: 38924095
-
Development of a Full-Thickness Human Skin Equivalent In Vitro Model Derived from TERT-Immortalized Keratinocytes and Fibroblasts.Tissue Eng Part A. 2015 Sep;21(17-18):2448-59. doi: 10.1089/ten.TEA.2015.0139. Epub 2015 Aug 3. Tissue Eng Part A. 2015. PMID: 26135533 Free PMC article.
-
Requisite instruments for the establishment of three-dimensional epidermal human skin equivalents-A methods review.Exp Dermatol. 2023 Nov;32(11):1870-1883. doi: 10.1111/exd.14911. Epub 2023 Aug 22. Exp Dermatol. 2023. PMID: 37605856 Review.
-
Recent advances in the epidermal growth factor receptor/ligand system biology on skin homeostasis and keratinocyte stem cell regulation.J Dermatol Sci. 2013 Nov;72(2):81-6. doi: 10.1016/j.jdermsci.2013.05.009. Epub 2013 Jun 13. J Dermatol Sci. 2013. PMID: 23819985 Review.
Cited by
-
A Systematic Review of Stem Cell Differentiation into Keratinocytes for Regenerative Applications.Stem Cell Rev Rep. 2024 Jan;20(1):362-393. doi: 10.1007/s12015-023-10636-9. Epub 2023 Nov 3. Stem Cell Rev Rep. 2024. PMID: 37922106
-
Genetic Tools for Cell Lineage Tracing and Profiling Developmental Trajectories in the Skin.J Invest Dermatol. 2024 May;144(5):936-949. doi: 10.1016/j.jid.2024.02.006. J Invest Dermatol. 2024. PMID: 38643988 Review.
References
-
- Kolarsick PAJ, Kolarsick MA, and Goodwin C. (2011). Anatomy and physiology of the skin. J. Dermatol. Nurses Assoc 3, 203–213. 10.1097/JDN.0b013e3182274a98. - DOI
Publication types
MeSH terms
Grants and funding
LinkOut - more resources
Full Text Sources
Molecular Biology Databases
Miscellaneous