Non-cell-autonomous activation of IL-6/STAT3 signaling mediates FGF19-driven hepatocarcinogenesis
- PMID: 28508871
- PMCID: PMC5440856
- DOI: 10.1038/ncomms15433
Non-cell-autonomous activation of IL-6/STAT3 signaling mediates FGF19-driven hepatocarcinogenesis
Abstract
Hepatocellular carcinoma (HCC), a primary malignancy of the liver, is the second leading cause of cancer mortality worldwide. Fibroblast Growth Factor 19 (FGF19) is one of the most frequently amplified genes in HCC patients. Moreover, mice expressing an FGF19 transgene have been shown to develop HCC. However, the downstream signalling pathways that mediate FGF19-dependent tumorigenesis remain to be deciphered. Here we show that FGF19 triggers a previously unsuspected, non-cell-autonomous program to activate STAT3 signalling in hepatocytes through IL-6 produced in the liver microenvironment. We show that the hepatocyte-specific deletion of Stat3, genetic ablation of Il6, treatment with a neutralizing anti-IL-6 antibody or administration of a small-molecule JAK inhibitor, abolishes FGF19-induced tumorigenesis, while the regulatory functions of FGF19 in bile acid, glucose and energy metabolism remain intact. Collectively, these data reveal a key role for the IL-6/STAT3 axis in potentiating FGF19-driven HCC in mice, a finding which may have translational relevance in HCC pathogenesis.
Conflict of interest statement
All authors are employees and stockholders of NGM Biopharmaceuticals, Inc.
Figures
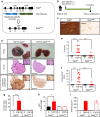


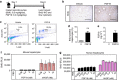
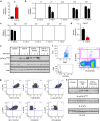


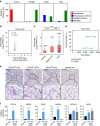
Similar articles
-
Regulation of amphiregulin gene expression by β-catenin signaling in human hepatocellular carcinoma cells: a novel crosstalk between FGF19 and the EGFR system.PLoS One. 2012;7(12):e52711. doi: 10.1371/journal.pone.0052711. Epub 2012 Dec 20. PLoS One. 2012. PMID: 23285165 Free PMC article.
-
Separating Tumorigenicity from Bile Acid Regulatory Activity for Endocrine Hormone FGF19.Cancer Res. 2014 Jun 15;74(12):3306-16. doi: 10.1158/0008-5472.CAN-14-0208. Epub 2014 Apr 11. Cancer Res. 2014. PMID: 24728076
-
FGF19 Protects Hepatocellular Carcinoma Cells against Endoplasmic Reticulum Stress via Activation of FGFR4-GSK3β-Nrf2 Signaling.Cancer Res. 2017 Nov 15;77(22):6215-6225. doi: 10.1158/0008-5472.CAN-17-2039. Epub 2017 Sep 26. Cancer Res. 2017. PMID: 28951455
-
Targeted inhibition of the FGF19-FGFR4 pathway in hepatocellular carcinoma; translational safety considerations.Liver Int. 2014 Jul;34(6):e1-9. doi: 10.1111/liv.12462. Epub 2014 Jan 24. Liver Int. 2014. PMID: 24393342 Review.
-
The Role of Fibroblast Growth Factor 19 in Hepatocellular Carcinoma.Am J Pathol. 2021 Jul;191(7):1180-1192. doi: 10.1016/j.ajpath.2021.04.014. Epub 2021 May 14. Am J Pathol. 2021. PMID: 34000282 Free PMC article. Review.
Cited by
-
Team players in the pathogenesis of metabolic dysfunctions-associated steatotic liver disease: The basis of development of pharmacotherapy.World J Gastrointest Pathophysiol. 2024 Aug 22;15(4):93606. doi: 10.4291/wjgp.v15.i4.93606. World J Gastrointest Pathophysiol. 2024. PMID: 39220834 Free PMC article. Review.
-
PPAR-γ/NF-kB/AQP3 axis in M2 macrophage orchestrates lung adenocarcinoma progression by upregulating IL-6.Cell Death Dis. 2024 Jul 26;15(7):532. doi: 10.1038/s41419-024-06919-9. Cell Death Dis. 2024. PMID: 39060229 Free PMC article.
-
New advances in drug development for metabolic dysfunction-associated diseases and alcohol-associated liver disease.Cell Biosci. 2024 Jul 6;14(1):90. doi: 10.1186/s13578-024-01267-9. Cell Biosci. 2024. PMID: 38971765 Free PMC article. Review.
-
Fibroblast growth factor signaling in macrophage polarization: impact on health and diseases.Front Immunol. 2024 Jun 19;15:1390453. doi: 10.3389/fimmu.2024.1390453. eCollection 2024. Front Immunol. 2024. PMID: 38962005 Free PMC article. Review.
-
MASLD treatment-a shift in the paradigm is imminent.Front Med (Lausanne). 2023 Dec 11;10:1316284. doi: 10.3389/fmed.2023.1316284. eCollection 2023. Front Med (Lausanne). 2023. PMID: 38146424 Free PMC article. Review.
References
-
- Llovet J. M. et al.. Hepatocellular carcinoma. Nat. Rev. Dis. Primers 2, 16018 (2016). - PubMed
-
- El-Serag H. B. Hepatocellular carcinoma. N. Engl. J. Med. 365, 1118–1127 (2011). - PubMed
-
- Michelotti G. A., Machado M. V. & Diehl A. M. NAFLD, NASH and liver cancer. Nat. Rev. Gastroenterol. Hepatol. 10, 656–665 (2013). - PubMed
-
- Zucman-Rossi J., Villanueva A., Nault J. C. & Llovet J. M. Genetic landscape and biomarkers of hepatocellular carcinoma. Gastroenterology 149, 1226–1239 e1224 (2015). - PubMed
Publication types
MeSH terms
Substances
LinkOut - more resources
Full Text Sources
Other Literature Sources
Medical
Molecular Biology Databases
Miscellaneous